Giménez-López
Liñas de investigación
Multifunctional Metal-Carbon Hybrid Nanostructures for Spintronics and Energy-related Applications
ERC - StG "NANOCOMP": Complex Dynamics of Clusters in High-Aspect Ratio Hollow Nanostructures
Investigador(es) principal/principais
Membros do grupo
Kumar, Satendra |
Inv. Posdoutoral |
|
Mácia Agulló, Juan Antonio |
Inv. Posdoutoral |
|
Sanchis Perucho, Adrián |
Inv. Posdoutoral |
|
Tayyebi, Ahmad |
Inv. Posdoutoral |
|
Medina Aguilar, Garoe |
Inv. Predoutoral |
|
Osorio Celis, Marcelo |
Inv. Predoutoral |
|
Varela Domínguez, Noa |
Inv. Predoutoral |
|
Vizcaíno Anaya, Lucía |
Inv. Predoutoral |
|
Rochette, Sophie |
Persoal técnico |
Investigación
Welcome to the research site of FunNanoMat Lab @CiQUS (USC). If you wish to have some Fun working or collaborating with our group, please contact us by e-mail. Funding opportunities arise frequently, so if you are interested please get in touch and we will get back to you soon.
Gimenez´s group has carried out a number of pioneering works with hybrid metal-carbon nanostructures, and the research is focused on the development and functional characterisation of hybrid nanostructures for spintronics and energy-related applications:
-
Assembling magnetic, electrical and electrochemical active nanoswitches within carbon nano-containers.
-
Developing a general synthetic methodology for the confinement of molecules, clusters and nanoparticles, setting a precedent in the field of nanomaterials chemistry.
Our research has yielded an entirely new class of hybrid nanostructures that can function as model systems to study frontier-computing concepts (e.g., quantum computing, spintronics), and develop new energy concepts in energy-storage and conversion. Our work has also pinpointed the effects of the unprecedented confinement of nanoswitches in carbon nanocontainers and vice versa.
As a result, Dr. Gimenez obtained the prestigous ERC- Starting Grant to develop her project "NANOCOMP: Complex Dynamics of Clusters in High-Aspect Ratio Hollow Nanostructures: A Nanoscale Platform for High-Performance Computing".
Encapsulation of single-molecule magnets in carbon nanotubes
This is the first example of “Encapsulation of single-molecule magnets in carbon nanotubes“ (Nature Communications 2011, 2:47) yielding a new type of hybrid nanostructure that combines all the key single-molecule magnet properties of the guest molecules with the functional properties of the host carbon nanotube.
This work has set a precedent in the field of molecular magnetism and spintronics, changing the way researches harness single-molecule magnets (SMM) properties and opening new avenues for their practical applications. Key results are:
-
Development of a successful methodology allowing transportation of intact single-molecule magnets (SMM) into nanotubes (CNT), which are very delicated molecules.
-
Demonstration of the key magnetic properties of SMM within CNT that is a very important result for construction of electronic devices based on these SMM-CNT hybrid materials.
Controlled Assembly and Magnetic Bistability of Mn3O4 Nanoparticles Encapsulated in Hollow Carbon Nanofibers
A key result in this work (Angew. Chem. Int. Ed. 2013, 52, 2051) is the demonstration of the precise control on the density and the orientation of the encapsulated magnetic nanoparticles in carbon nanofibers by the internal structure of the host-nanocontainer, which enables tuning functional magnetic properties of the composite material. Through Van der Waals forces the interiors of corrugated carbon nanofibers, acting as a site for strong interaction between nanofibers and nanoparticles, disrupt assemblies in magnetic nanoparticles.
It opens up a number of exciting opportunities for applications requiring the precise control of the position and the orientation of guest-nanoparticles, for example, nanoelectronics.
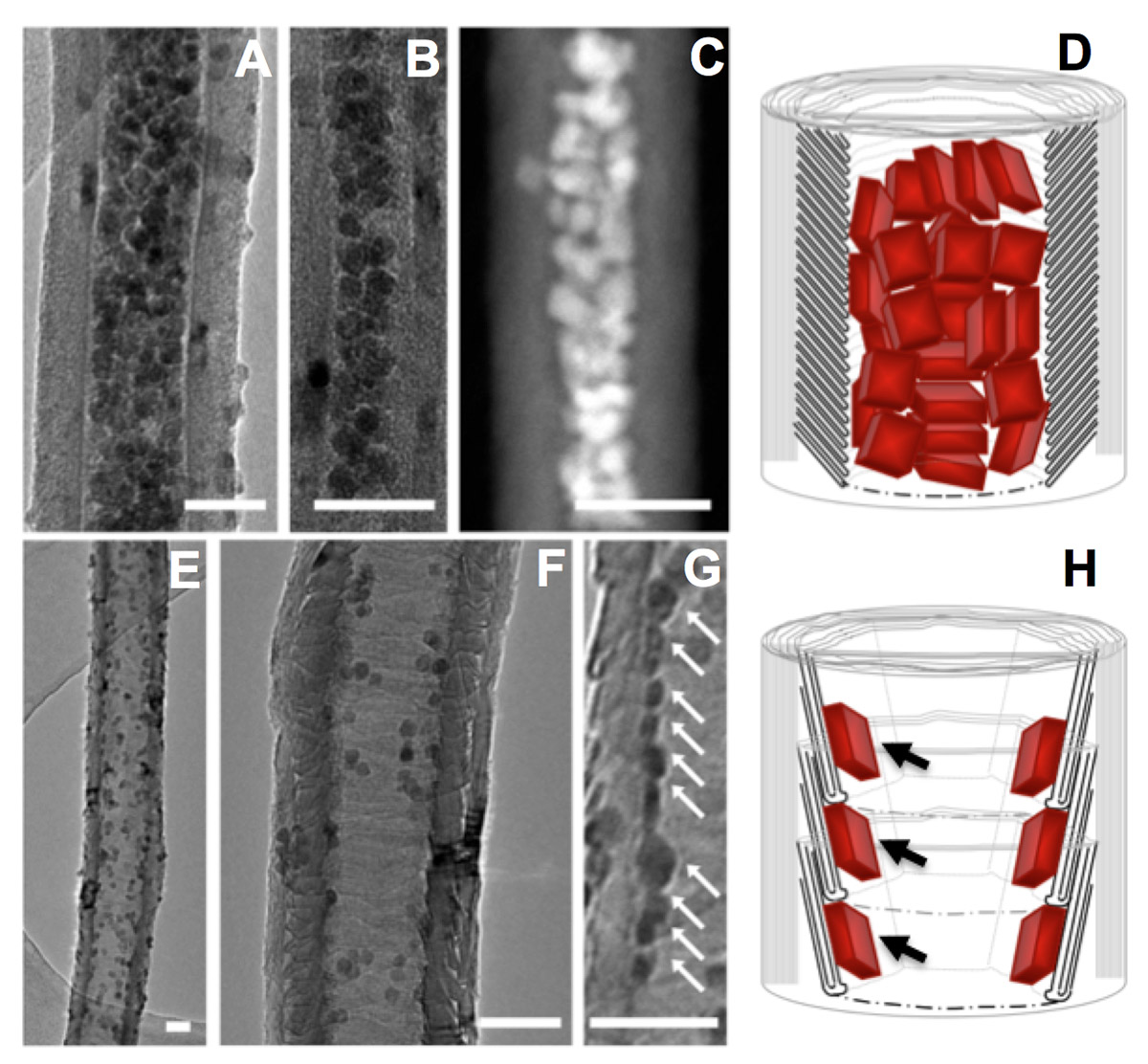
Dense packing of NP in Mn3O4@CNF, as revealed by bright field TEM (A-B) and high-angle annular dark-field scanning TEM (HAADF STEM) (C).
Bright-field TEM images of Mn3O4 nanobricks adsorbed on the step-edges of GNF (E–G). The scale bars are 40 nm.
Dynamics of Gold Nanoparticles on Carbon Nanostructures Driven by van der Waals and Electrostatic Interactions
We have developed a pioneering methodology (Small, 2015, 11, 23, 2756), for trapping and developing metallic nanoparticles at the one-dimensional nanochannels formed between a cylindrical carbon nanotube and a flat surface (few layers graphene) to form highly ordered, stable heterostructures.
In this work the control of the position and the size of the adsorbed nanoparticles at temperatures as high as 500 °C when few layers graphene is used as flat support is a very important breakthrough for potential application of these hybrid nanomaterials. This work demonstrates the relative importance of van der Waals forces and electrostatic interactions, for the creation and stabilisation of highly ordered, linear arrangements of metallic nanoparticles of controlled size.
These findings offer a powerful route for the development of novel electrocatalyst materials for energy-related and synthesis applications, spintronics devices with improved thermal stability, and will enhance functional performance of metastable nanostructures in general, in the pursuit of stable, well-organised systems.
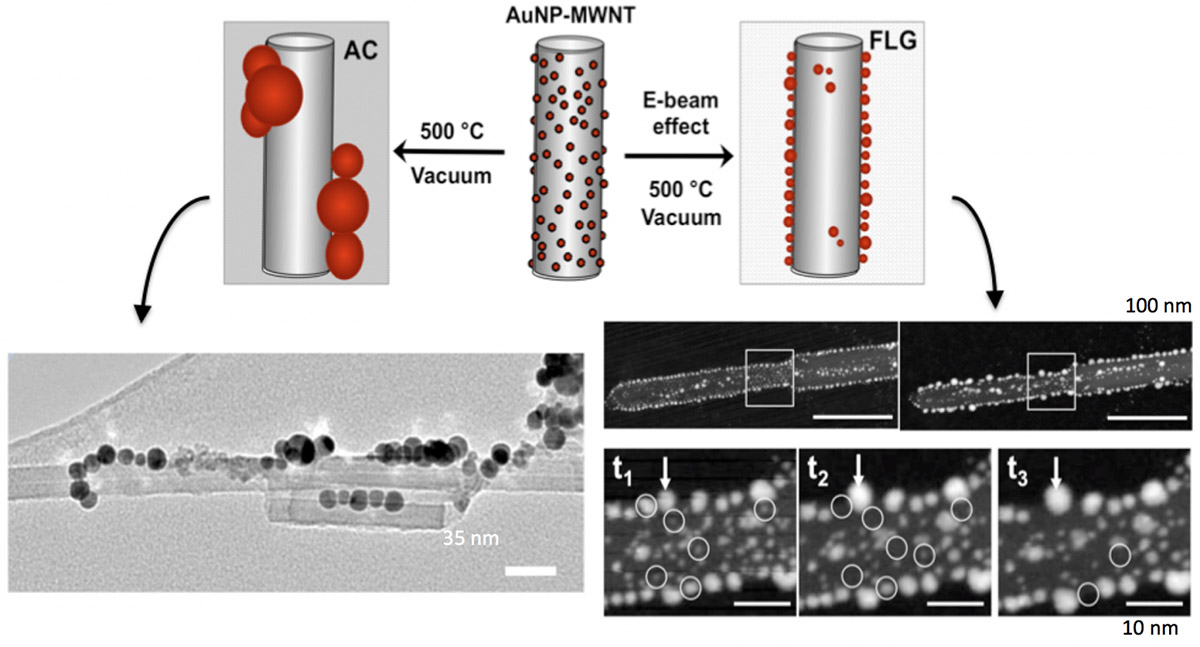
Extremely Stable Platinum-Amorphous Carbon Electrocatalyst within Hollow Graphitized Carbon Nanofibers for the Oxygen Reduction Reaction
In this ground-breaking study
(Adv. Mater., 2016, 28, 41, 9103, inside back cover), we have employed hollow carbon nanofibers that can act simultaneously as nanoscale containers (anchoring catalytically active Pt nanoparticles) and nano-electrodes (providing an effective electric bridge between the nanoparticles and the macroscopic electrode).
For the first time, the crucial role of nanographene step-edges present in the carbon nanofibers interiors in retaining the catalytic activity of the anchored Pt nanoparticles and preventing undesired processes, such as coalescence, electromigration and Ostwald ripening that are responsible of the durability problems has been directly evidenced. It has been demonstrated Pt nanoparticles encased within graphitic nanofibers as very effective catalysts in the electrochemical reaction of oxygen reduction, which forms a basis of the fuel cells technology.
This novel hybrid nanocatalyst shows performance comparable to commercial electrocatalysts (Pt/carbon black), but most importantly it exhibits outstanding durability, retaining most of the electrocatalytic activity even after 50,000 cycles of the reaction, and thus significantly outperforming all existing electrocatalytic systems under these conditions.
This unprecedented discovery illustrates that the hybrid nanocatalyst is self-stabilising during the use, which contrasts to the usual catalysts behaviour. It is important both on the fundamental scientific level, as it sheds light on the nanoscale processes under electrocatalytic conditions, and on the applied level, as it offers a new strategy to reduce the use of precious metals such as Pt in electrochemical devices by prolonging their lifetime.
Figure 4. Comparison of PtNP@S-GNF and Pt/C over 50 k potential cycles for (a) the specific activity (SA) and (b) the electrochemical active surface area (ECSA). TEM images of PtNP@S-GNF before (c) and after cycling (d), and schematic representation of our catalyst for the ORR.